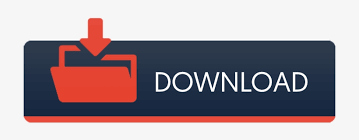
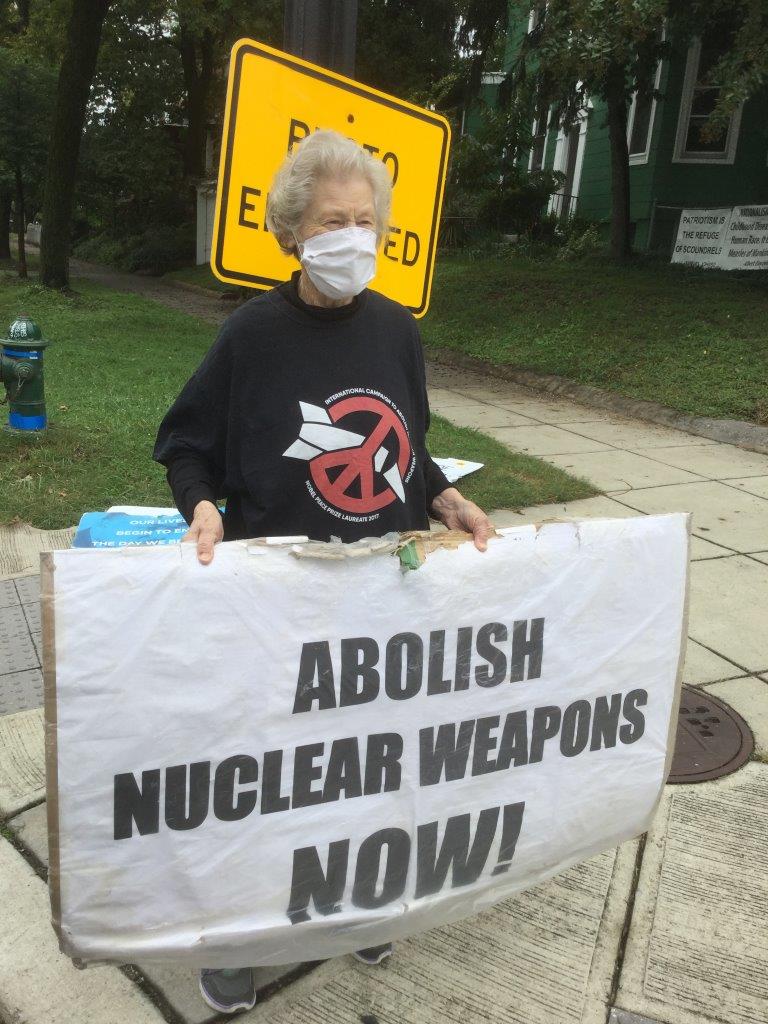
Both are taken up through the food cycle and readily incorporated in organic matter. To the extent that hydrogen fusion contributes to the explosive force of a weapon, two other radionuclides will be released: tritium (hydrogen-3), an electron emitter with a half-life of 12 years, and carbon-14, an electron emitter with a half-life of 5,730 years. Plutonium-239 decays through emission of an alpha particle (helium nucleus) and has a half-life of 24,000 years. A bone-seeker like strontium-90, it may also become lodged in the lungs, where its intense local radiation can cause cancer or other damage. In addition, there is plutonium-239, frequently used in nuclear explosives. Iodine-131 is a similar threat to infants and children because of its concentration in the thyroid gland. Strontium-90 follows calcium chemistry, so that it is readily incorporated into the bones and teeth, particularly of young children who have received milk from cows consuming contaminated forage.
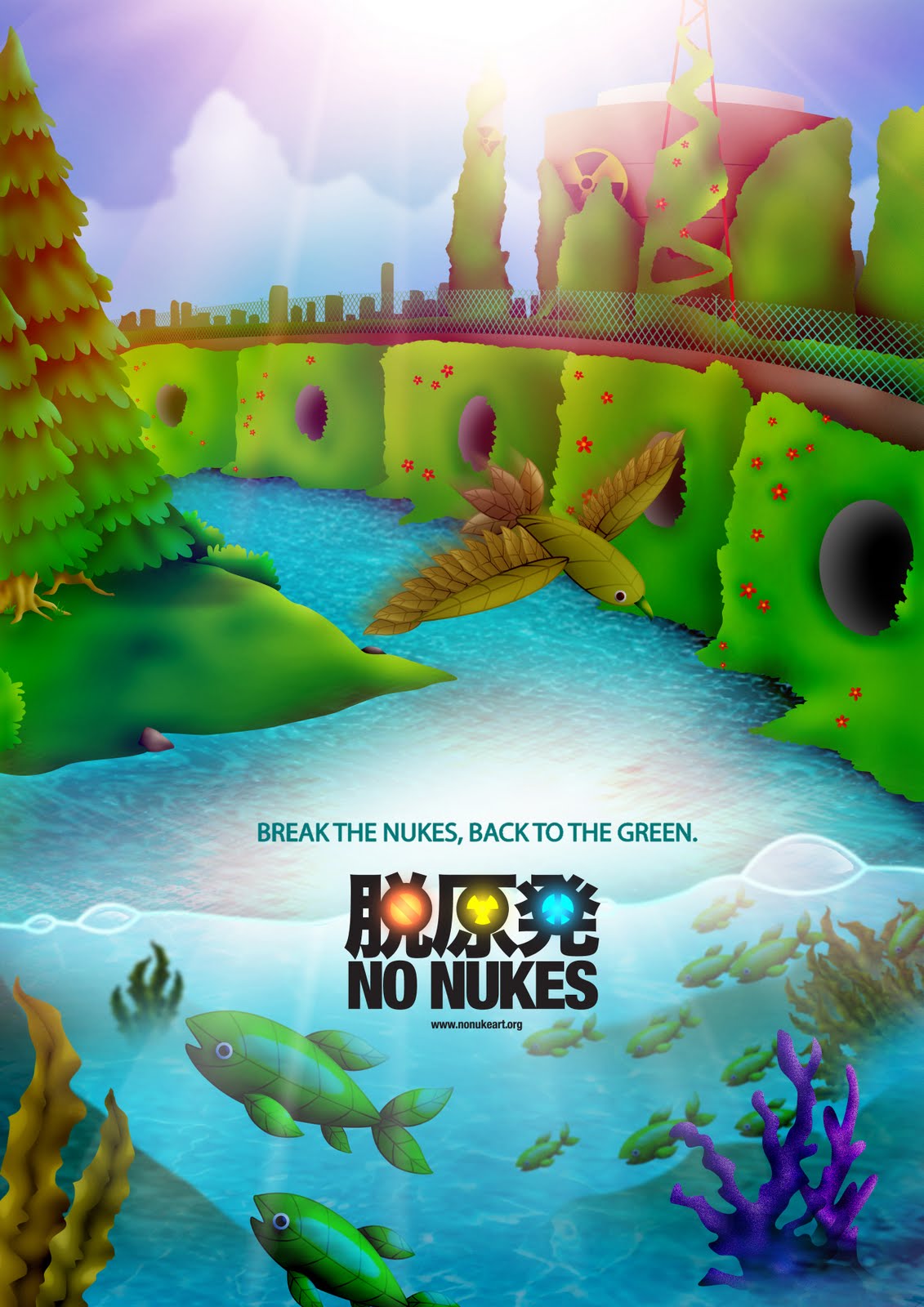
Other hazards are strontium-90, an electron emitter with a half-life of 28 years, and iodine-131 with a half-life of only 8 days. It is a major source of radiation in nuclear fallout, and since it parallels potassium chemistry, it is readily taken into the blood of animals and men and may be incorporated into tissue. Probably the most serious threat is cesium-137, a gamma emitter with a half-life of 30 years. If this occurs, the risk of biological damage from the destructive ionizing radiation (see "Radioactivity" note) is multiplied. This determines whether they will be taken up by the body through respiration or the food cycle and incorporated into tissue. (See "Nuclear Half-Life" note.) Another factor which is critical in determining the hazard of radionuclides is the chemistry of the atoms. This is measured in terms of "half-life"-the time required for one-half of the original substance to decay-which ranges from days to thousands of years for the bomb-produced radionuclides of principal interest. (See "Radioactivity" note.) An important characteristic here is the rate of decay. The nuclear fragments of heavy-element fission which are of greatest concern are those radioactive atoms (also called radionuclides) which decay by emitting energetic electrons or gamma particles. Thus, all nuclear detonations produce radioactive fragments of heavy elements fission, with the larger bursts producing an additional radiation component from the fusion process. However, the fission process is still necessary to achieve the high temperatures and pressures needed to trigger the hydrogen fusion reactions. Since there is virtually no limitation on the volume of fusion materials in a weapon, and the materials are less costly than fissionable materials, the fusion, "thermonuclear," or "hydrogen" bomb brought a radical increase in the explosive power of weapons. The larger yield nuclear weapons derive a substantial part of their explosive force from the fusion of heavy forms of hydrogen-deuterium and tritium. The smaller nuclear weapon, in the low-kiloton range, may rely solely on the energy released by the fission process, as did the first bombs which devastated Hiroshima and Nagasaki in 1945.
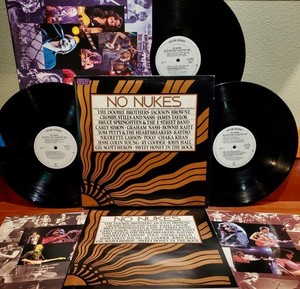
The energy released in this fission process is many millions of times greater, pound for pound, than the most energetic chemical reactions. Both the local and worldwide fallout hazards of nuclear explosions depend on a variety of interacting factors: weapon design, explosive force, altitude and latitude of detonation, time of year, and local weather conditions.Īll present nuclear weapon designs require the splitting of heavy elements like uranium and plutonium.
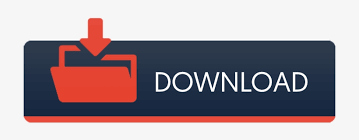